النبات
مواضيع عامة في علم النبات
الجذور - السيقان - الأوراق
النباتات الوعائية واللاوعائية
البذور (مغطاة البذور - عاريات البذور)
الطحالب
النباتات الطبية
الحيوان
مواضيع عامة في علم الحيوان
علم التشريح
التنوع الإحيائي
البايلوجيا الخلوية
الأحياء المجهرية
البكتيريا
الفطريات
الطفيليات
الفايروسات
علم الأمراض
الاورام
الامراض الوراثية
الامراض المناعية
الامراض المدارية
اضطرابات الدورة الدموية
مواضيع عامة في علم الامراض
الحشرات
التقانة الإحيائية
مواضيع عامة في التقانة الإحيائية
التقنية الحيوية المكروبية
التقنية الحيوية والميكروبات
الفعاليات الحيوية
وراثة الاحياء المجهرية
تصنيف الاحياء المجهرية
الاحياء المجهرية في الطبيعة
أيض الاجهاد
التقنية الحيوية والبيئة
التقنية الحيوية والطب
التقنية الحيوية والزراعة
التقنية الحيوية والصناعة
التقنية الحيوية والطاقة
البحار والطحالب الصغيرة
عزل البروتين
هندسة الجينات
التقنية الحياتية النانوية
مفاهيم التقنية الحيوية النانوية
التراكيب النانوية والمجاهر المستخدمة في رؤيتها
تصنيع وتخليق المواد النانوية
تطبيقات التقنية النانوية والحيوية النانوية
الرقائق والمتحسسات الحيوية
المصفوفات المجهرية وحاسوب الدنا
اللقاحات
البيئة والتلوث
علم الأجنة
اعضاء التكاثر وتشكل الاعراس
الاخصاب
التشطر
العصيبة وتشكل الجسيدات
تشكل اللواحق الجنينية
تكون المعيدة وظهور الطبقات الجنينية
مقدمة لعلم الاجنة
الأحياء الجزيئي
مواضيع عامة في الاحياء الجزيئي
علم وظائف الأعضاء
الغدد
مواضيع عامة في الغدد
الغدد الصم و هرموناتها
الجسم تحت السريري
الغدة النخامية
الغدة الكظرية
الغدة التناسلية
الغدة الدرقية والجار الدرقية
الغدة البنكرياسية
الغدة الصنوبرية
مواضيع عامة في علم وظائف الاعضاء
الخلية الحيوانية
الجهاز العصبي
أعضاء الحس
الجهاز العضلي
السوائل الجسمية
الجهاز الدوري والليمف
الجهاز التنفسي
الجهاز الهضمي
الجهاز البولي
المضادات الحيوية
مواضيع عامة في المضادات الحيوية
مضادات البكتيريا
مضادات الفطريات
مضادات الطفيليات
مضادات الفايروسات
علم الخلية
الوراثة
الأحياء العامة
المناعة
التحليلات المرضية
الكيمياء الحيوية
مواضيع متنوعة أخرى
الانزيمات
Speciation
المؤلف:
AN INTRODUCTION TO PLANT BIOLOGY-1998
المصدر:
JAMES D. MAUSETH
الجزء والصفحة:
27-10-2016
2603
Speciation
As natural selection operates on a population for many generations, the frequencies of various alleles and consequently the phenotype of the population change. At some point, so much change has occurred that the current population must be considered a new species, distinct from the species that existed at the beginning. Natural selection has caused a new species to evolve, a process called speciation.
At what point can we conclude that a new species exists? It is not possible to give an exact definition of species that is always valid, but generally, two organisms are considered to be members of distinct species if they do not produce fertile offspring when crossed. Many exceptions exist, however. The individuals of maples in the western United States cannot naturally reproduce sexually with those in the eastern United States, but that is only because the pollen does not travel that far; if an eastern plant is brought close to a western one artificially, they can cross-fertilize and are therefore considered the same species. On the other hand, many orchid species grow together in nature without producing hybrids, but artificial cross-breeding may produce healthy, fertile hybrids. Nevertheless, they are considered distinct species because they look different from each other. So, if two plants freely interbreed in nature, they are members of the same species; if they do not interbreed even when manually cross-pollinated they are separate species; and if they do not interbreed naturally but they do artificially, it is a judgment call on the part of the scientists who work with the plants and are most familiar with them.
Speciation can occur in two fundamental ways: (1) phyletic speciation, in which ore species gradually becomes so changed that it must be considered a new species (Fig. 1a). and b), and (2) divergent speciation, in which some populations of a species evolve into a new, second species while other populations either continue relatively unchanged as the original, parental species or evolve into a new, third species (Fig. 1c and d).
FIGURE 1: (a) and (b) In phyletic speciation, all of a species gradually changes because one particular aspect of a character is advantageous for all individuals. Here, all leaves become larger; perhaps the climate is becoming more humid or herbivorous insects are less of a problem. (c) and (d) In this scenario of divergent evolution, both extremes of the condition are more advantageous than intermediate values; the climate may become drier in some areas, favoring the smaller leaves, but moister in other areas, favoring plants with larger leaves.
PHYLETIC SPECIATION
Millions of years are often required for a species to evolve into a new one. The critical feature is that as new beneficial alleles arise and are selected for, they become spread throughout the entire population. This movement of alleles physically through space, called gene flow, occurs in many ways, such as by pollen transfer, seed dispersal, and vegetative propagation.
Pollen Transfer. Pollen grains each carry one full haploid genome, and all alleles of a plant are present in its pollen grains. Wind-distributed pollen, such as that of ragweed, grasses, and conifers, can travel great distances (Fig. 2). If a new allele is carried by some of the pollen grains, it can move to very distant plants; if the pollen grain's sperm cells fertilize an egg, a new seed is formed whose embryo contains the new allele.
Animal-mediated pollination also contributes to gene flow; both birds and insects lend to spend most of their time in a small area, so although birds can fly long distances, they usually spread pollen through a smaller area. Nevertheless, allele movement can be rapid.
FIGURE 2:Strong winds can blow pollen great distances, resulting in gene flow between widely separated points. Much of the pollen actually settles near the parent plant, but large numbers of pollen grains may travel a long distance and then successfully fertilize eggs. The new sporophytes pass on the pollen's genes to their progeny. Pinus canariensis. (Robert and Linda Mitchell).
Seed Dispersal. The fruits and seeds of some plants fall close to the parent, but many species have long-distance dispersal mechanisms. Seeds and fruits can be carried by wind, floods, and stream flow. They can be carried to islands by rafting, in which they are trapped above water on a tangled mat of floating debris. Seeds or fruits that are spiny or gummy stick to the fur or feathers of animals; migratory animals can be especially important in dispersing seeds. Most birds reduce their weight before migration by preening themselves of all adhesive seeds. But if just one seed is overlooked, its alleles are transferred; as the seed germinates, grows into a new plant, and reproduces, the new alleles can be spread throughout the new site.
Vegetative Propagation. If a species produces small, mobile pieces that reproduce vegetatively, these too contribute to gene flow.
If these various mechanisms are sufficient to enable alleles that arise in one part of the species' range to travel to all other parts, the species remains relatively homogeneous, even as the entire species evolves into a new species. Alleles that arise at various geographic sites ultimately come together by gene flow; then meiosis, crossing-over, and genetic recombination rearrange them into thousands of combinations (Fig. 3).
FIGURE 3:Gene flow. This range is almost divided in two by a series of mountains over which pollen and seeds cannot travel; this slows down gene flow and homogenization of the population. The numbers indicate the generation in which the allele flows: 1—gene flow in the first generation; 2—gene flow from plants that grew from seeds produced by the gene flow of the first generation, and so on. Here, three generations were necessary for the new allele to become distributed to the lower side of the mountains, seven generations to become widespread throughout the range. In a species of annual plants, each generation is 1 year long; for biennials, a generation is 2 years long and gene flow is slower. In trees that do not produce their first flowers, pollen, or seeds until they are many years old, gene flow can be very slow.
DIVERGENT SPECIATION
If gene flow does not keep the species homogeneous throughout its entire range, divergent speciation may occur; if alleles that arise in one part of the range do not reach individuals in another part, the two regions are reproductively isolated.
Reproductive isolation can occur in many ways, but the two fundamental causes are a biological and biological reproductive barriers.
A biological Reproductive Barriers. Any physical, nonliving feature that prevents two. populations from exchanging genes is an abiological reproductive barrier. The original species is physically divided into two or more populations that cannot interbreed; if speciation results, it is called allopatric speciation. Mountain ranges are frequently reproductive barriers because pollinators do not fly across entire mountain ranges while feeding or gathering pollen. Seeds may occasionally be carried across mountains by birds or mammals, but probably too rarely to be significant. Rivers are often good barriers for small animals, but they rarely prevent plant gene flow by means of seed dispersal. Deserts and oceans are effective barriers. Plants that are adapted to the harsh conditions of mountain tops are reproductively isolated from plants on adjacent mountain tops by the intervening valleys because pollinators and seed dispersers do not often travel from mountain to mountain. Any seeds dropped into the valley would not be able to compete with lowland plants and would die. Ultraviolet (UV) light and dry air are barriers to very long-distance wind dispersal of pollen; during the ride on the wind, pollen is damaged by the UV light and dry air and often dies before it can travel far.
Biological Reproductive Barriers. Any biological phenomenon that prevents successful gene flow is a biological reproductive barrier. Differences in flower color, shape, or fragrance can be effective barriers if the species is pollinated by a discriminating pollinator. A mutation that inhibits pigment synthesis and causes a normally colored plant to haw; white flowers might prevent the pollinator from recognizing the flower. The flower is not visited and gene flow no longer occurs between the mutant plant and the rest of the species even though the individuals grow together. Timing of [lowering can be important: If some; flowers open in the evening, they probably do not interbreed with those that open in the morning. Differences in flowering date arc critical because of the brief viability of pollen once it leaves the anthers. When two groups become reproductively isolated even though they grow together, the result is sympatric speciation.
Evolutionary changes in pollinators can also act as reproductive barriers for plants. If a plain population covers a large area, some parts of the range probably have characteristics different from those of other parts, such as elevation, temperature, and humidity. These variations may be important to pollinators and seed distributors if not to the plant. If so, the animals may diverge evolutionarily into distinct species, each limited to a small part of the plant's range. Little or no flow of the plant's genes occurs owing to the restricted movements of pollinators and seed dispersers. These examples of biological reproductive barriers prevent pollen from moving from one plant to another, so neither pollination not fertilization occurs. Consequently, these are called prezygotic isolation mechanisms: They act even before a zygote can be formed.
The environmental diversity of a large geographic range can lead directly to divergence of the plants themselves. Although a plant species may be able to occupy an extensive, heterogeneous range, with time mutations arise that are particularly adaptive for certain aspects of specific regions of the range. When these new alleles arrive at that part of the range by gene flow, their frequency there is increased by natural selection. When they arrive at other parts of the range, their frequency remains low or they are eliminated there if they are neutral or selectively disadvantageous for conditions in these sites (Fig. 4). Even with active gene flow and interbreeding, different subpopulations of the plant species emerge, each adapted to its own particular portion of the total range. As this process continues, each subpopulation becomes progressively more distinct and may be recognized and named as a subspecies. Finally, because of the large number of unique, charac- teristic alleles, each may become sufficiently distinct phenotypically that the two subpopulations can no longer interbreed: Their genomes are too different. At this point, postzygotic internal isolation barriers are in place, and the two subpopulations must be consider! separate species.
FIGURE 4:A mutation has produced an allele highly adaptive in dry parts of the range but neutral or deleterious in moist parts. Because it originated in a less-than-optimal environment, in generation 1 only two offspring survive. In generation 2, each of these produces two more that survive, one in a moister habitat (A), one in a drier region (B). The allele is deleterious at A, and only a single progeny survives in generation 3; at B, two survive, both in drier habitats, and in generation 4 each produces three surviving progeny. As the alleles flow, by chance, into regions that are more optimal (drier), reproductive success is greater and the allele frequency increases. As they flow, by chance, into unsuitable habitats, reproductive success is low and the allele never becomes abundant.
One of the earliest postzygotic barriers to arise is hybrid sterility: The two populations occasionally interbreed or are artificially cross-pollinated and produce viable seed, but the seed grows into a sterile plant. Spore mother cells in anthers and ovules in the sterile hybrid are unable to complete meiosis because of a failure of synapsis: "Homologouts chromosomes are no longer truly homologous, and they fail to form pairs and synaptonemal complexes during prophase I. Without fertile pollen or ovules, no seed is formed and the mixture of the two genomes ends when the hybrid plant dies. The two populations continue to diverge and become even more distinctly separate species. If cross-pollination occurs, alleles from one parent may code for proteins incompatible with those coded by alleles from the other, and not even a sterile hybrid can result; instead, the zygote or embryo dies early in development.
Once internal isolation barriers are established, evolutionary divergence should be even more rapid because new alleles cannot be shared; gene flow between the two populates comes to a stop. Each species becomes more easily recognizable as new characters evolve in each which are not present in its sibling species. The two species are initially so similar that they are obviously related; such closely related species are grouped together no genera by taxonomists.
Divergent evolution may result in numerous types of new species; in some cases, one subpopulation changes into a new species while the remaining part of the population continues relatively unchanged as the original species. In other cases, both subpopulations change so much that two new species emerge and the original species no longer exists. The original species has become extinct, although it has numerous progeny that form the members of two new species.
Active Radiation. Adaptive radiation is a special case of divergent evolution in which a species rapidly diverges into many new species over an extremely short time, just a few million years. This usually occurs when the species enters a new habitat where little or no competition or environmental stress exists. The best examples are the colonization of newly formed oceanic islands such as the Hawaiian or Galapagos Islands. After being formed by volcanic activity, they are initially devoid of all plant and animal life, but eventually a seed arrives, carried by either a bird, the wind, or ocean currents. Once the seed germinates and begins to grow, it is free of danger from herbivores, fungi, bacteria, or competition from other plants. It must be relatively adapted to the soil, rainfall pattern, and heat/cold fluctuations, but otherwise its life is remarkably free of dangers. If this plant is self-fertile or if it reproduces well vegetatively, it successfully colonizes the area.
All offspring greatly resemble the first, founder individual(s), because the initial gene pool is extremely small; if just one seed is the founder, the original gene pool consists of its two sets of alleles. This homogeneity may last only briefly because, with the lack of competition, pathogens, and predators, fewer forces act as selective agents. Consequently, new alleles build up in the population much more rapidly than can occur in the parental population on the mainland (Fig. 5). While the population is small, it is more subject to accidents, so the gene pool can change rapidly and erratically; this is genetic drift, and the island population soon becomes heterogeneous. Natural selection for adaptation to soil types, drainage, climate, and metabolism is still operative, so divergence is based largely on physical factors in the environment.
FIGURE 5:One of the pioneer plants that arrived and colonized the Hawaiian Islands was a composite, related to daisies. Because of the low levels of competition and because so few individuals existed initially, each mutation became a significant part of the gene pool even while only one or a few copies existed, so genetic drift was rapid. Argyroxyphium, silver swords, grow only in Haleakala National Park in Hawaii. Goats ate them almost to extinction before the park was established. (Stephen ]. Krasemann/© Peter Arnold, Inc.)
If the island is very far from any seed source (the mainland or other islands), like Hawaii is, hundreds of years may pass before the arrival of more seeds of either the same or another species. Even if it is relatively close to the mainland, like the Galapagos, seeds arrive only rarely. For thousands of years the only animals might be birds or airborne insects, typically spiders; land reptiles (lizards) and mammals (mice) arrive by rafting, but this can be extremely rare.
Adaptive radiation can also occur in mainland populations if the environment changes suddenly and eliminates the dominant species of a region. With the absence of these species, competition changes and other species that had not been able to compete well before can now occupy the new areas; while few in number, they undergo genetic drift, rapidly producing many unusual genotypes. Within a short time, many new species are recognizable, and adaptive radiation has occurred.
CONVERGENT EVOLUTION
If two distinct, unrelated species occupy the same or similar habitats, natural selection my favor the same phenotypes in each. As a consequence, the two may evolve to the point that they resemble each other strongly and are said to have undergone convergent evolution. The most striking example is the evolutionary convergence of cacti and euphorbias (Figs. Inc.) 1b, 6 and 7). Cacti evolved from leafy trees in the Americas; as deserts formed, mutations that prevented leaf formation were advantageous because they reduced transpiration. Other selectively advantageous mutations increased water storage capacity (succulent trunk) and defenses against water-seeking animals (spines). In Africa, the formation of deserts also favored a similar phenotype, and the euphorbia family became adapted by these means. As a result, the succulent euphorbias are remarkably similar to the succulent cacti, even though the ancestral euphorbias are quite distinct from the ancestral cacti Two groups cannot converge to the point of producing the same species; only the phenotypes converge, not the genotypes. For example, cactus spines are modified leaves, whereas euphorbia spines are modified shoots.
FIGURE 6:Euphorbia gymnocalycioides, often mistaken for a cactus. Careful examination shows that many anatomical features are also different. (Courtesy of Ron LaFon).
FIGURE 7:Cacti and euphorbias have undergone extensive convergent evolution, and each is parasitized by distinct types of mistletoe that have also undergone convergent evolution. Viscum minimum infects Euphorbia lactea; compare it with Tristerix aphyllus, which infects the cactus Trichocereus chilensis. The selective advantages associated with T. aphyllus and its succulent, desert-adapted host were discussed in Box 5.1. Those same considerations are also valid for Viscum minimum and Euphorbia lactea.
الاكثر قراءة في مواضيع عامة في علم النبات
اخر الاخبار
اخبار العتبة العباسية المقدسة
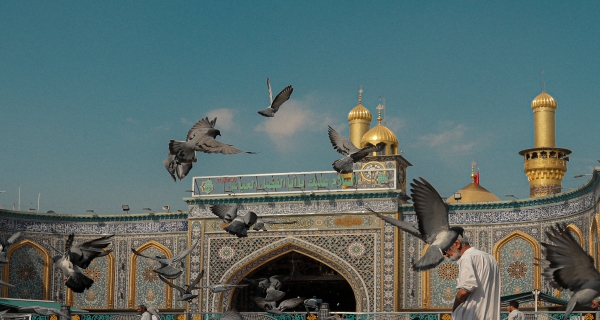
الآخبار الصحية
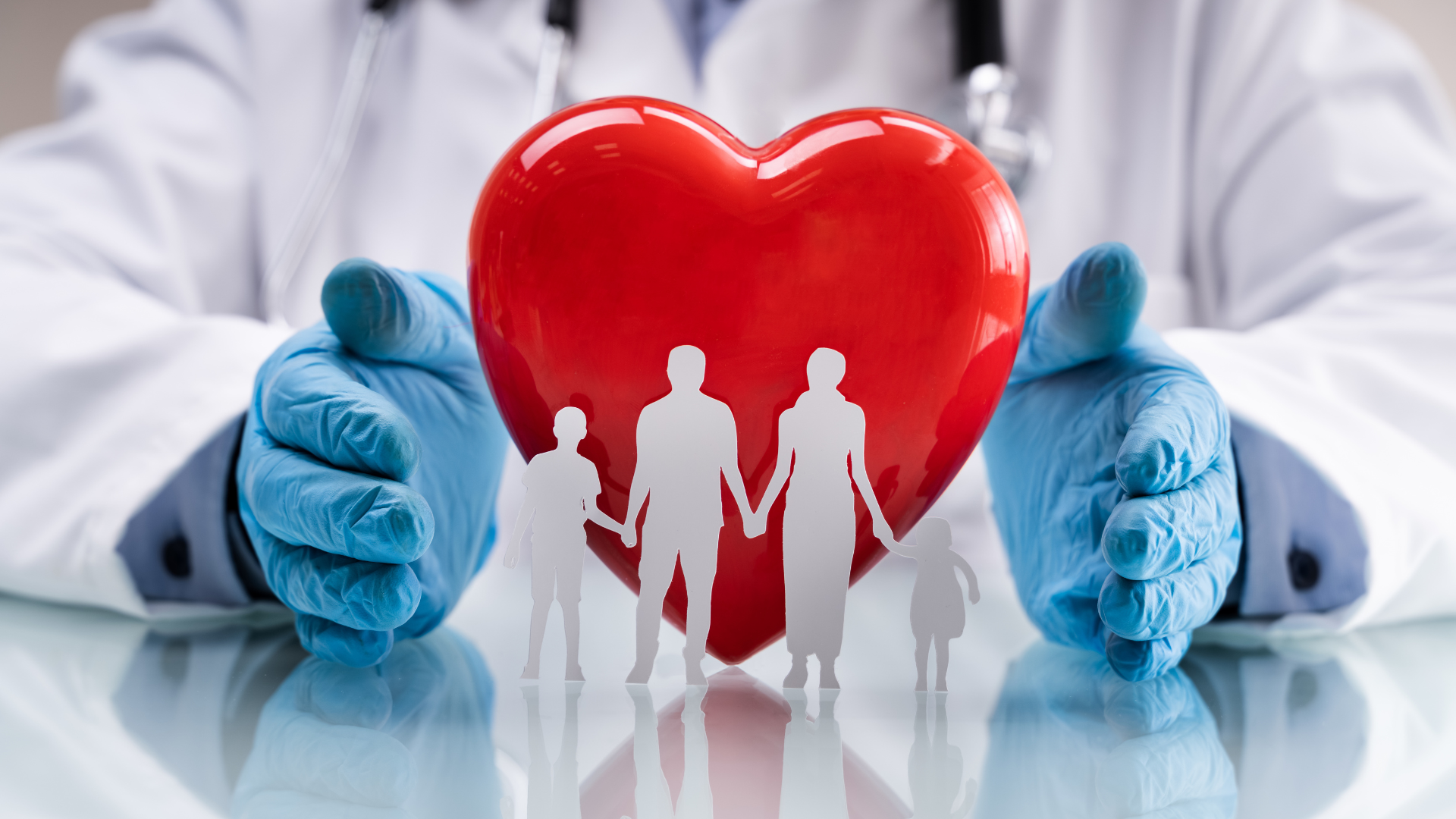