النبات
مواضيع عامة في علم النبات
الجذور - السيقان - الأوراق
النباتات الوعائية واللاوعائية
البذور (مغطاة البذور - عاريات البذور)
الطحالب
النباتات الطبية
الحيوان
مواضيع عامة في علم الحيوان
علم التشريح
التنوع الإحيائي
البايلوجيا الخلوية
الأحياء المجهرية
البكتيريا
الفطريات
الطفيليات
الفايروسات
علم الأمراض
الاورام
الامراض الوراثية
الامراض المناعية
الامراض المدارية
اضطرابات الدورة الدموية
مواضيع عامة في علم الامراض
الحشرات
التقانة الإحيائية
مواضيع عامة في التقانة الإحيائية
التقنية الحيوية المكروبية
التقنية الحيوية والميكروبات
الفعاليات الحيوية
وراثة الاحياء المجهرية
تصنيف الاحياء المجهرية
الاحياء المجهرية في الطبيعة
أيض الاجهاد
التقنية الحيوية والبيئة
التقنية الحيوية والطب
التقنية الحيوية والزراعة
التقنية الحيوية والصناعة
التقنية الحيوية والطاقة
البحار والطحالب الصغيرة
عزل البروتين
هندسة الجينات
التقنية الحياتية النانوية
مفاهيم التقنية الحيوية النانوية
التراكيب النانوية والمجاهر المستخدمة في رؤيتها
تصنيع وتخليق المواد النانوية
تطبيقات التقنية النانوية والحيوية النانوية
الرقائق والمتحسسات الحيوية
المصفوفات المجهرية وحاسوب الدنا
اللقاحات
البيئة والتلوث
علم الأجنة
اعضاء التكاثر وتشكل الاعراس
الاخصاب
التشطر
العصيبة وتشكل الجسيدات
تشكل اللواحق الجنينية
تكون المعيدة وظهور الطبقات الجنينية
مقدمة لعلم الاجنة
الأحياء الجزيئي
مواضيع عامة في الاحياء الجزيئي
علم وظائف الأعضاء
الغدد
مواضيع عامة في الغدد
الغدد الصم و هرموناتها
الجسم تحت السريري
الغدة النخامية
الغدة الكظرية
الغدة التناسلية
الغدة الدرقية والجار الدرقية
الغدة البنكرياسية
الغدة الصنوبرية
مواضيع عامة في علم وظائف الاعضاء
الخلية الحيوانية
الجهاز العصبي
أعضاء الحس
الجهاز العضلي
السوائل الجسمية
الجهاز الدوري والليمف
الجهاز التنفسي
الجهاز الهضمي
الجهاز البولي
المضادات الحيوية
مواضيع عامة في المضادات الحيوية
مضادات البكتيريا
مضادات الفطريات
مضادات الطفيليات
مضادات الفايروسات
علم الخلية
الوراثة
الأحياء العامة
المناعة
التحليلات المرضية
الكيمياء الحيوية
مواضيع متنوعة أخرى
الانزيمات
Ion-exchange chromatography
المؤلف:
Clive Dennison
المصدر:
A guide to protein isolation
الجزء والصفحة:
18-4-2016
2922
Ion-exchange chromatography (IEC)
Consider the situation where a single anion type, say CH3COO-, and a number of cation types, say Na+, K+ and Protein+, exist together in an aqueous solution, where the aqueous component will additionally contribute OH- and H+ ions. These ions will establish a dynamic equilibrium, comprised of a number of sub-equilibria, e.g.
The overall equilibrium condition will be determined by the values of the respective dissociation constants:-
The overall equilibrium state is a result of-
• intrinsic affinities between ions (expressed in the dissociation
constants), and,
• competition between ions (a function of the relative concentrations of the ions).
To develop a chromatography system, the CH3COO- ion, in the form of a carboxymethyl group (-CH2COO-), could be covalently attached to the stationary phase and the dissociated cations allowed to move with the mobile phase: net electrical neutrality being maintained, however. With an immobilized anion, this would constitute a cation exchange system Conversely, immobilization of a cation would constitute an anion exchange system.
If the above ions were applied to the chromatography system as a sample “plug” and the system was subsequently eluted with a buffer, say lithium citrate, the differential affinities of the ions for the stationary anion would be manifest as differential rates of migration through the column. The ions would migrate at relative rates proportional to their respective dissociation constants. Their manifest affinity, and thus their absolute rates of migration, would depend upon the competition that they encountered from the buffer cation, Li+ in this example. With increasing Li+ concentration, the sample cations would face increasing competition in their association with the immobilized anion and so would be increasingly dissociated, resulting in an increase in their rate of migration through the column. In the case of proteins, the dissociation constant is affected by pH, so elution can also be effected by a change in pH.
1 . Ion-exchange “resins”
The term “resin” comes from early polystyrene-based ion-exchangers which had a translucent yellow appearance, like the resin exudates from pine trees. The term has stuck, although modern ion-exchangers used for protein separations are generally opaque and white.
All ion-exchange resins are comprised of a matrix to which are attached ionic substituent groups. For low pressure chromatography of proteins, the matrix is often comprised of a hydrophilic biopolymer, such as cellulose, Sephadex™, or agarose. These materials cannot withstand high pressures and for medium to high pressure liquid chromatography, the trend is towards silica-based resins, or synthetics such as Trisacryl ™.
Cellulose is a polymer of ß-D-glucose units, linked with bonds. It is relatively inexpensive and provides good flow properties, but large interstitial spaces lead to relatively poor resolution. Sephadex consists of dextran chains, comprised of linked dextrose (glucose) residues, cross-linked with epichlorhydrin. The name “Sephadex” is a contraction of the words “separating”, “Pharmacia” and “dextran”. It is sold in the form of dry xerogels which absorb water and swell into hydrated spherical particles. Substituted Sephadex ion-exchangers give good resolution but they are subject to marked volume changes with changes in buffer ionic strength. This is a disadvantage as it is difficult to apply an accurate salt gradient to a shrinking gel, and it may become necessary to re-pack the column after only a few runs.
Agarose is the neutral polysaccharide component of agar, an extract of kelp, which is a type of seaweed. It is a linear polysaccharide composed of alternating residues of D-galactose and 3,6-anhydro-L galactose, linked by →3 and→ 4 bonds.
Figure 1. The structure of agarose.
Agarose is freely soluble in water at 100°C and upon cooling forms an exceptionally strong, so-called macroreticular gel, with large pores (Fig. 2).
Figure 2. Comparison of micro- and macroreticular gels.
Sephadex (Fig. 2A) is an example of a microreticular gel. In a macroreticular gel (Fig. 2B), e.g. agarose, the gel fibres align into bundles resulting in a much stronger gel and a larger pore size at a given gel concentration. The sketch in Fig. 2 is a 2-D representation, but gels actually form 3-D labyrinths.
The macroreticular structure of agarose makes it very suitable as a matrix for ion-exchangers as proteins have easy access to the gel interior, so that in effect the gel has a very large surface area to which ionic substituent groups may be attached. The macroreticular structure is also mechanically strong, so that substituted agarose ion-exchangers do not shrink or swell with changes in buffer ionic strength. The gel structure of agarose is maintained by non-covalent bonds and agarose gels cannot be dried and reconstituted. They are consequently supplied in the form of a slurry. They also cannot be boiled or autoclaved, as they simply melt to a sol at high temperatures.
Common substituent groups are shown in Table 1. The common weak base anion exchanger group is DEAE- and the common weak acid cation exchanger group is CM-. The pH range over which these groups are ionized is shown in Fig. 3.
Table 1. Some common ion-exchange substituent groups.
Figure 3. Ionization characteristics of CM- and DEAE- substituent groups.
It can be seen from Fig. 65 that exchangers comprised of weak acid or weak base substituent groups are not completely ionized at most pH values of interest and, perhaps a greater drawback, the degree of ionization changes with pH in the useful pH range. Exchangers based on strong acids or bases, by contrast, are Completely ionized over a much larger pH range, so their degree of ionization is less subject to change with pH (Fig. 4).
Figure 4. Ionization of strong acid and strong base ion-exchange substituent groups.
2 . Gradient generators
Ion-exchange chromatography often requires elution of the bound proteins by a change in either ionic strength, pH, or both. If the system being separated is well characterized, then appropriate stepwise changes can be made, An example is in the ion-exchange separation of amino acids in an amino acid analyzer. More commonly, in protein isolation the exact characteristics of the components being separated are unknown and it is then necessary to use a gradient generator to effect an ionic strength or pH gradient.
Gradients are commonly generated in one of two ways. A two- chamber device, with a magnetic stirrer stirring one chamber, may be used, but an even simpler arrangement is to have two conical flasks with a siphon arranged between them (Fig. 5).
Figure 5. A simple gradient generator set-up.
The starting solution is placed in the right hand vessel and the finishing solution in the left hand vessel. A siphon is established between
The vessels by sucking solution up and clamping the T-piece side tubing. The rigid tubing can consist of flexible tubing inserted inside of, for e.g., plastic disposable pipettes.
A more sophisticated, but more expensive, method of generating a gradient is to use a micro-processor controlled proportioning valve which draws liquid alternately from one vessel and then the other, in small amounts which gradually change in proportion with time. A mixer is placed in the line, downstream of the proportioning valve, to change the small stepwise changes in buffer composition into a gradual and continuous change. Gradient generators based on proportioning valves are common components of complete chromatography systems, commercially available from a number of manufacturers.
3 . Choosing the pH
One of the decisions that has to be made before conducting ion- exchange chromatography is what pH to use. The selection of pH and of the type of ion-exchanger to use may be facilitated by establishing the so-called titration curves of the proteins in the mixture to be separated. An electrophoretic titration curve can be determined by establishing a pH gradient in a gel and conducting an electrophoretic separation at right-angles to the pH gradient (Fig. 6).
Figure 6. Electrophoretic titration curves of proteins.
In Fig. 6, pH “a” is the pH of maximal charge difference between proteins A and B. At this relatively low pH, both proteins have a positive charge and a cathodic migration. This information suggests that cation-exchange chromatography, conducted at pH “a”, would most likely effect the best separation between proteins A and B. By contrast, at pH “d” the proteins have no apparent charge difference. This implies that anion-exchange chromatography would be less successful at resolving A and B, especially at pH “d”. Points “b” and “c” represent the pI values of proteins B and A, respectively. The difference in these pI values gives an indication of the separation which may be expected from isoelectric focusing or Chromatofocusing.
4 . An ion-exchange chromatography run
The choice of the type of exchanger, cation- or anion-exchanger, may be arbitrary, in the absence of any knowledge of the characteristics of the protein of interest, e.g. its pI and/or its pH stability range. As a first approach, it is generally best to choose a pH, within the protein’s stability range, where it will adsorb to the ion-exchanger. Usually, this means that an anion-exchanger should be used at pH values above the pI of the protein and a cation-exchanger at a pI below the pI. (It must be realized, however, that the pI refers to an overall property of the protein, whereas binding to an ion-exchanger is a function of the charge on one surface of the protein. It is therefore possible to have a protein bind to an anion-exchanger at a pH below its pI or to a cation-exchanger at a pH above its pI. It becomes a matter of experimentally exploring the behaviour of each new unknown protein.)
With the resin chosen and the column packed, it is necessary to equilibrate the column with several column volumes (“colvols”) of starting buffer, a buffer of low ionic strength and of a pH which will promote binding of the protein of interest to the resin. The sample protein mixture must contain a low salt concentration, achieved by equilibrating it with the starting buffer; either by dialysis, ultrafiltration, molecular exclusion chromatography or, following TPP, by simply re- dissolving the precipitate in the starting buffer.
The sample solution is applied to the column and chased with at least 2 colvols of starting buffer in order to elute the unbound fraction. The A280 may be monitored during this process and elution with starting buffer stopped once the A280 returns to the baseline. At this point a buffer gradient may be applied. As a first approach, a gradient of increasing ionic strength is the best choice, and is applicable to both cation- and anion-exchange.
The resolution of peaks is a function of the steepness of the eluting gradient. A shallow gradient gives better resolution, but takes more time, so a trade-off must be made. With an unknown system, the best first approach is to use a steep gradient, as this gives a quick assessment of the number of peaks to be expected, and the separation can subsequently be optimized. A suitably steep gradient for a first approach is M NaCl in starting buffer, in 3 colvols. For subsequent optimization the gradient limits or the number of colvols can be altered, to change the gradient slope.
It must be appreciated that the gradient is applied to the inlet side of the column, whereas monitoring of the effluent is done on the outlet side. There is thus a colvol difference between the influent and effluent streams, Consequently, after application of the gradient, it is necessary to elute with at least one colvol of finishing buffer to ensure that the whole gradient itself is eluted and that all peaks which would be eluted by the gradient are, in fact, washed from the column. An example of the reporting of an ion-exchange chromatography run is presented in Fig. 7.
Figure 7. An example of ion-exchange chromatography : Purification of cathepsin S, from a TPP fraction from bovine spleen, by chromatography on S-Sepharose. Column, 2.5 an i.d. x 17 cm; Starting buffer, 20 inM Na-acetate containing 1 mM EDTA and 0.02% NaN3, pH 5.0; Gradient, 0------300 in M NaCl in 600 ml. (Note that the column is eluted with 1 column volume of finishing buffer after the gradient is applied, in order to elute proteins which may have been displaced by the buffer but not yet eluted from the column.) The thick line indicates cathepsin S activity and the thin line is A280. (The apparent activity in the break-through peak reflects the fact that the assay is not absolutely specific for cathepsin S).
S-Sepharose is a cation exchanger. If an anion exchanger were used, elution with an ionic strength gradient could be effected in exactly the same way. A different strategy is needed for cation and anion exchangers, however, if a pH gradient is used to elute the bound proteins. In the case of a cation exchanger, a gradient of rising pH is required, whereas with an anion exchanger a gradient of descending pH is required. After completion of the chromatography run, with either a cation or anion exchanger, firmly bound sample components may be eluted with a high salt concentration, such as 1 M NaCl. In the case of cation exchangers, high salt concentration may be combined with a high pH, and with anion exchangers the high salt may be combined with a low pH. The extreme pH values used must, of course, be within the stability limits of the ion-exchanger resin. Finally, the column is re-equilibrated with several colvols of starting buffer, in preparation for the next run.
Ion-exchange chromatography thus requires cycling through large changes in ionic strength and possibly also of pH. As mentioned previously it is an advantage if the resin can withstand the required ionic strength and pH changes, without shrinking or swelling, as this makes it possible to cycle through the changes in buffer composition without having to repack the column.
References
-Dennison, C. (2002). A guide to protein isolation . School of Molecular mid Cellular Biosciences, University of Natal . Kluwer Academic Publishers new york, Boston, Dordrecht, London, Moscow .
الاكثر قراءة في عزل البروتين
اخر الاخبار
اخبار العتبة العباسية المقدسة
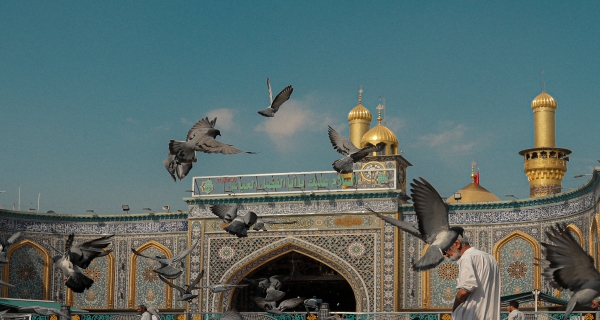
الآخبار الصحية
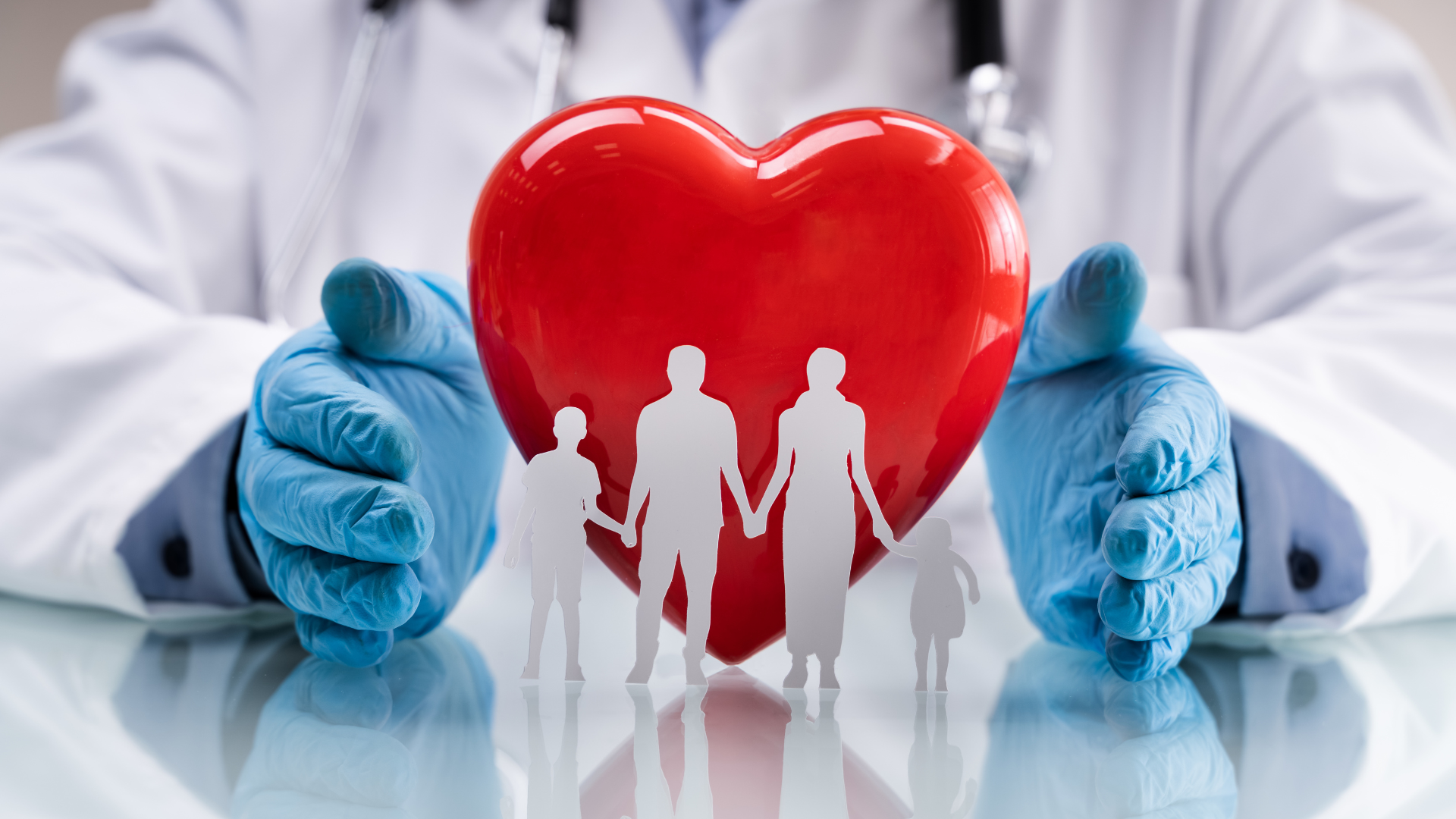