تاريخ الفيزياء
علماء الفيزياء
الفيزياء الكلاسيكية
الميكانيك
الديناميكا الحرارية
الكهربائية والمغناطيسية
الكهربائية
المغناطيسية
الكهرومغناطيسية
علم البصريات
تاريخ علم البصريات
الضوء
مواضيع عامة في علم البصريات
الصوت
الفيزياء الحديثة
النظرية النسبية
النظرية النسبية الخاصة
النظرية النسبية العامة
مواضيع عامة في النظرية النسبية
ميكانيكا الكم
الفيزياء الذرية
الفيزياء الجزيئية
الفيزياء النووية
مواضيع عامة في الفيزياء النووية
النشاط الاشعاعي
فيزياء الحالة الصلبة
الموصلات
أشباه الموصلات
العوازل
مواضيع عامة في الفيزياء الصلبة
فيزياء الجوامد
الليزر
أنواع الليزر
بعض تطبيقات الليزر
مواضيع عامة في الليزر
علم الفلك
تاريخ وعلماء علم الفلك
الثقوب السوداء
المجموعة الشمسية
الشمس
كوكب عطارد
كوكب الزهرة
كوكب الأرض
كوكب المريخ
كوكب المشتري
كوكب زحل
كوكب أورانوس
كوكب نبتون
كوكب بلوتو
القمر
كواكب ومواضيع اخرى
مواضيع عامة في علم الفلك
النجوم
البلازما
الألكترونيات
خواص المادة
الطاقة البديلة
الطاقة الشمسية
مواضيع عامة في الطاقة البديلة
المد والجزر
فيزياء الجسيمات
الفيزياء والعلوم الأخرى
الفيزياء الكيميائية
الفيزياء الرياضية
الفيزياء الحيوية
الفيزياء العامة
مواضيع عامة في الفيزياء
تجارب فيزيائية
مصطلحات وتعاريف فيزيائية
وحدات القياس الفيزيائية
طرائف الفيزياء
مواضيع اخرى
The Unruh Density Matrix
المؤلف:
Leonard Susskind And James Lindesay
المصدر:
AN INTRODUCTION TO BLACK HOLES, INFORMATION, AND THE STRING THEORY REVOLUTION
الجزء والصفحة:
14-12-2015
2511
The Unruh Density Matrix
Now let us consider the space of states describing a Lorentz invariant quantum field theory in Minkowski space. In Figure 1.1 the surface T = 0 of Minkowski space is shown divided into two halves, one in Region I and one in Region III.F or the case of a scalar field χ the fields at each point
Fig. 1.1. Fields on the spacelike surface T = 0 in Minkowski space.
of space form a complete set of commuting operators. These fields may be decomposed into two subsets associated with regions I and III. we call the χR and χL respectively. Thus
(1.1)
The general wave functional of the system is a functional of χL and χR
Ψ = Ψ(χL, χR) (1.2)
We wish to compute the density matrix used by the Fidos in Region I to describe their Rindler world. In particular we would like to understand the density matrix ρR which represents the usual Minkowski vacuum to the Fidos in Region I.
First let us see what we can learn from general principles. Obviously the state Ψ is translationally invariant under the usual Minkowski space translations Th us the Fidos must see the vacuum as invariant under translations along the X and Y axes. However, the translation invariance along the Z axis is explicitly broken by the act of singling out the origin Z = 0 for special consideration. From the X, Y translation invariance we conclude that ρR commutes with the components of momentum in these directions
(1.3)
A very important property of ρR is that it is invariant under Rindler time translations ω → ω + constant. This follows from the Lorentz boost invariance of Ψ. Thus
(1.4)
To proceed further we must use the fact that Ψ(χL, χR) is the ground state of the Minkowski Hamiltonian. General path integral methods may be brought to bare on the computation of the ground state wave functional. Let us assume that the field theory is described in terms of an action
(1.5)
The so-called Euclidean field theory is defined by replacing the time coordinate T by iX0. For example, the Euclidean version of ordinary scalar field theory is obtained from the usual Minkowski action
(1.6)
Letting T → iX0 we obtain the Euclidean action
(1.7)
Now a standard method of computing the ground state by path integration is to use the Feynman–Hellman theorem. Suppose we wish to compute Ψ(χL, χR).Then we consider the path integral
(1.8)
where the path integral is over all χ(x) with X0 > 0 and Z is an appropriate normalization factor .The field χ(x) is constrained to equal (χL, χR) on the surface X0 = 0. Finally the action IE is evaluated as an integral over the portion of Minkowski space with X0 > 0.
The boost invariance of the original Minkowski action insures that the Euclidean action has four dimensional rotation invariance. In particular, the invariance under ω-translations becomes invariance under rotations in the Euclidean (Z,X0) plane. This suggests a new way to carry out the path integral. Let us define the Euclidean angle in the (Z,X0) plane to be θ. The angle θ is the Euclidean analogue of the Rindler time ω. Now let us divide the region X0 > 0 into infinitesimal angular wedges as shown in Figure 1.2.
Fig. 1.2. Euclidean analogue of Rindler space for path integration
The strategy for computing the path integral is to integrate over the fields in the first wedge between θ = 0 and θ = δθ. The process can be iterated until the entire region X0 > 0 has been covered.
The integral over the first wedge is defined by constaining the fields a θ = 0 and θ = δθ. This defines a transfer matrix G in the Hilbert space of the field configuration χR. The matrix is recognized to be
G = (1 − δθHR) . (1.9)
To compute the full path integral we raise the matrix G to the power π/δω giving
(1.10)
In other words, the path integral defining Ψ is computed as a transition matrix element between initial state χR and final state χL. The infinitesimal generator which pushes θ surfaces forward is just the Rindler Hamiltonian. Now we are prepared to compute the density matrix ρR. The density matrix ρR is given by
(1.11)
Now using equation 1.10 we get
(1.12)
In other words, the density matrix is given by the operator
(1.13)
This remarkable result, discovered by William Unruh in 1976 , says that the Fidos see the vacuum as a thermal ensemble with a density matrix of the Maxwell–Boltzmann type. The temperature of the ensemble is
(1.14)
The derivation of the thermal character of the density matrix and the value of the Rindler temperature in equation 1.14 is entirely independent of the particulars of the relativistic field theory. It is equally correct for a free scalar quantum field, quantum electrodynamics, or quantum chromodynamics.
الاكثر قراءة في الثقوب السوداء
اخر الاخبار
اخبار العتبة العباسية المقدسة
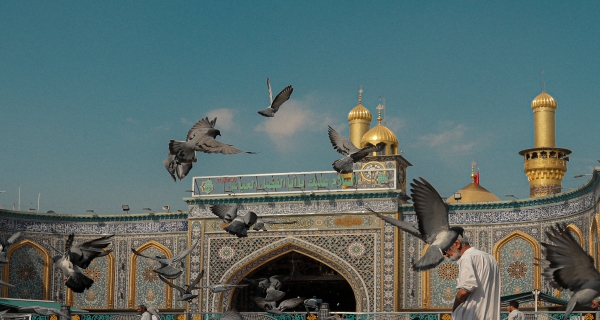
الآخبار الصحية
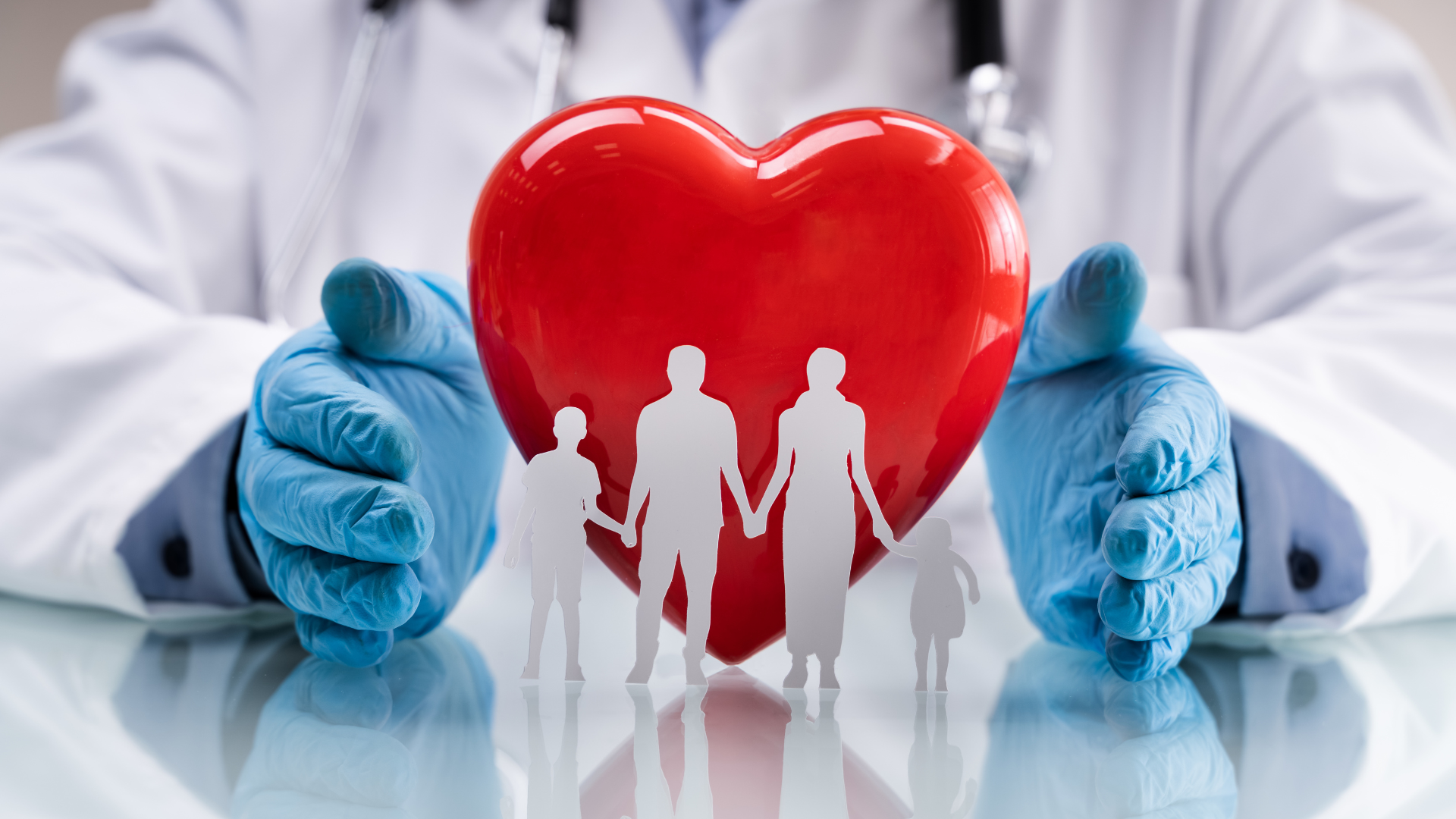